Abstract
As a widely used group of alloys, Mg-Li alloys are improved through treatments including precipitation hardening, solution strengthening, grain and sub-grain optimization, and dislocation density strengthening.
Solution strengthening for example, is one of the most effective mechanisms used to improve the mechanical properties of Mg-Li alloys and its composites and through a series of studies we explore the results.
Mg-Li based alloys are widely applied in various engineering applications. The strength of these alloys is modified and enhanced by different strengthening mechanisms. The strengthening mechanisms of these alloys and their composites have been extensively studied during the past decades. Important mechanisms applied to strengthening the alloys include precipitation strengthening, solution strengthening, grain and subgrain strengthening, and dislocation density strengthening.
Modeling Strengthening Mechanisms in Mg-Li Based Alloys and Composites
Precipitation Strengthening. In binary systems, alloying above a specific concentration given by the phase diagram causes the formation of a second phase. A second phase can also be created through mechanical or thermal treatment methods. The second phase precipitates act as pinning points in a similar manner to solutes. The precipitation strengthening effect in Mg-Li alloys depends on the size, morphology, distribution, and volume fraction of the precipitates and the nature of the interface between the precipitate and the matrix formed which can be coherent or incoherent.
Solution Strengthening. Solution strengthening is one of the most effective mechanisms used to improve the mechanical properties of Mg-Li alloy and its composites. This strengthening mechanism is scientifically explained from both elastic and electronic origins. The elastic origin is quantitatively justified based on the impurity-dislocation interaction atomic theory. The atomic size factor greatly contributes to the strengthening mechanism because it strengthens Mg-Li alloy phases due to dissolved atoms. Solid solution strengthening (𝜎ss) occurs because the atoms in the solution alter and change lattice parameters and atomic binding forces of the alloys. In Mg-Li alloys and composites, the dissolved atoms effectively interact with dislocations and twinning, leading to the enhancement of strength.
The article of D. Kuc, E. Hadasik, A. Mrugala presents the results of tests connected with influence of strain parameters on the susceptibility to plastic shaping of magnesium lithium alloys with lithium content of 4.0, 7.5% and 15% of mass. Plasticity tests were conducted in compression test from room temperature up to 400°C with strain speed of 0.1s-1. Conducted tests allowed for determination of susceptibility of magnesium alloys with different lithium content to plastic shaping. The results were compared with typical alloy AZ31. The aim of the paper was to compare the plasticity of magnesium alloy AZ31 with alloy content from 4 to 15% lithium.
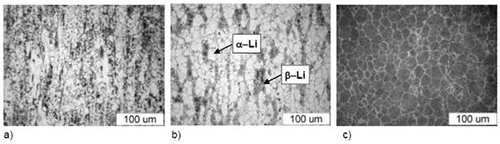
Figure 1: Microstructure of Mg-Li alloys in initial state after casting and hot extrusion: a – Mg4%Li, b – Mg-7.5%Li and c – 15%Li alloy (dark field)
At room temperature in alloy AZ31 the failure occurs with strain value of 0.2. With lithium content of 4.0% and 7,5% the failure occurs with strain value of about 0.3 and 0,4 respectively (Figures 2 - 4). Increase of deformation temperature to 100°C leads to improvement of formability all alloys do not reach the given deformation. Given strain is achieved by alloys AZ31 and Mg-4%Li in temperature of 200°C, and alloy containing 7.5% lithium reaches it in 150°C. Together with the increase of lithium content regardless of strain temperature the value of maximum flow stress σp decreases in comparison with alloy AZ31. In temperature of 300°C for Mg-4%Li alloy the maximum value of σp equals 90 MPa (Figure 2), and by 7.5% Li it drops to value of 60 MPa (Figure 4). Flow stress-strain curves of alloy AZ31 are characteristic for alloy in which during deformation a mechanism of plastic strain called twinning occurs. As it can be observed, the decrease of deformation temperature, below 250°C changes the shape of flow curve for tested alloys with 4.0% and 7,5% lithium. A curve is achieved which initially has concave shape, which is connected with intensive course of twinning in microstructure (Figure 5a). The presence of twinning mechanism in deformation of magnesium alloys decreases with the increase of lithium content and by big amounts of lithium (7,5% Li) it is practically observed from room temperature to 150°C. Itis visible in this range that the most beneficial susceptibility to deformation is alloy Mg -15 %Li (Figure 4). The alloy deformed in the temperature from room temperature to 300°C demonstrate the most advantageous susceptibility to plastic forming from investigated alloy.
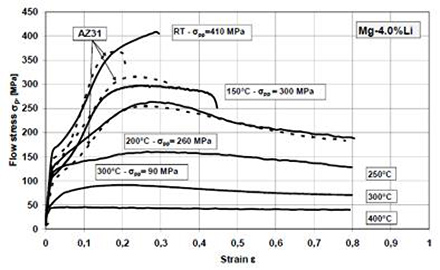
Figure 2: Results of compression test of magnesium alloy AZ31 and Mg-4%Li at temperature range from room temperature (RT) to 400°C
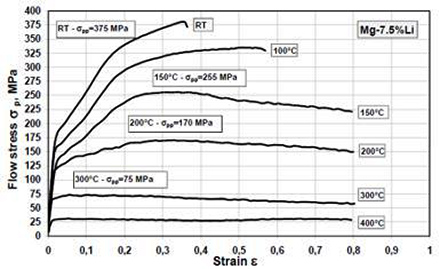
Figure 3: Results of compression test of Mg-7,5%Li alloy at temperature range from room temperature (RT) to 400°C
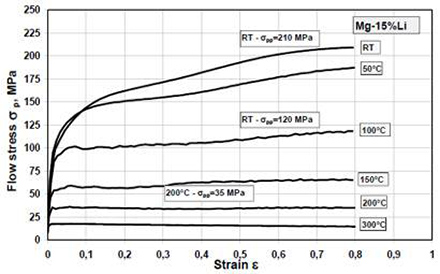
Figure 4: Results of compression test of a Mg-15%Li alloy at temperature range from room temperature (RT) to 300°C
Compression of samples in low temperature leads to formation of a lot of mechanical twins of deformation in the microstructure (Figure 5a). A rise in the compression temperature to 300°C leads to the occurrence of fine dynamically recrystallized grains on primary grain boundaries (Figure 5b). Deformation at 400°C leads to intensive recrystallization of the structure (Figure 6). At deformation temperature of 400°C a completely dynamically recrystallized structure become apparent bot both Mg-4,0%Li and Mg-7,5%Li. For compression microstructure of Mg-15%Li alloy is fully recrystallized after compression in temperature of 300°C.
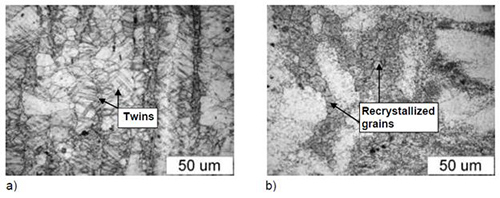
Figure 5: Microstructure of Mg-7,5% Li alloys after hot deformation at temperature 100°C to strain ε = 0.58 and Mg-4,0% Li after deformation at temperature 300°C to strain ε = 0.8
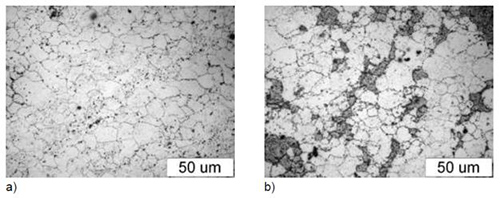
Figure 6: Microstructure of Mg-4% Li alloy (a) and Mg-7,5%Li alloy (b) after hot deformation at temperature 400°C to strain ε = 0.8